Endogenous tagging using split mNeonGreen in human iPSCs for live imaging studies
Figures
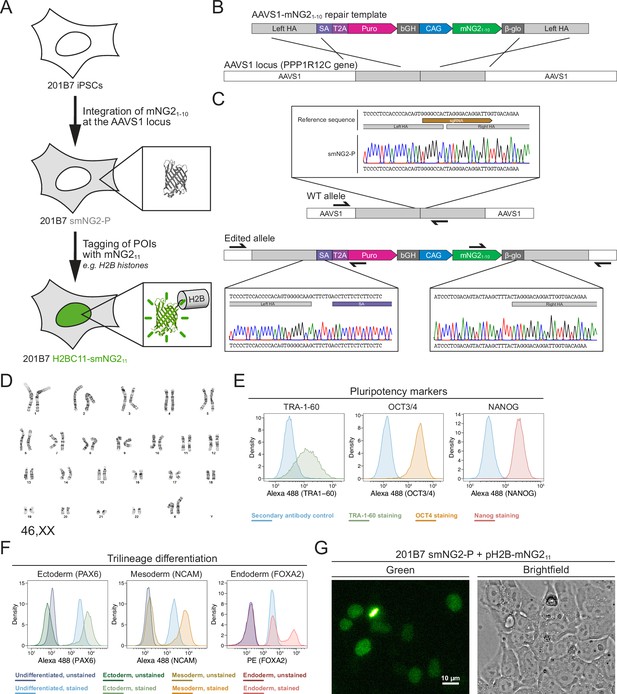
Generation of a split mNeonGreen human induced pluripotent stem (iPS) cell line.
(A) A schematic representation of the split tagging strategy used in this study. An expression cassette carrying the mNG21-10 fragment was integrated at the AAVS1 locus in 201B7 iPSCs to generate the parental split mNeonGreen2 cell line (smNG2-P). Proteins of interest were tagged endogenously with the mNG211 fragment to visualize their expression and localization. The structure of mNeonGreen was generated from PDB (Protein Data Bank, structure identifier 5 LTP; Clavel et al., 2016). (B) A schematic of the mNG21-10 expression cassette is shown with the CAG promoter (blue) and sequences for stable expression (dark gray), Puromycin resistance marker (pink), and homology arms (light gray) for integration at the AAVS1 locus. Abbreviations: SA = splicing acceptor, T2A=Thosea asigna virus 2A peptide, bGH = bovine growth hormone poly-adenylation signal, β-glo=rabbit β-globin poly-adenylation signal. (C) Sequencing chromatograms show the edited AAVS1 allele junctions in the smNG2-P cell line (bottom) compared to the WT allele (top). (D) Representative G-banding karyotype of the smNG2-P cell line shows that the edited cells have a normal karyotype (46, XX). (E) Flow cytometry plots show smNG2-P cells stained for pluripotency markers TRA-1–60 (left, green), OCT3/4 (center, yellow), and NANOG (right, red) or a secondary antibody control (blue). (F) Flow cytometry plots show differentiated smNG2-P cells stained for PAX6 (ectoderm; left, green), NCAM (mesoderm; center, yellow), and FOXA2 (endoderm; right, red) compared to undifferentiated cells (blue) and unstained controls (dark colors). (G) Fluorescent and brightfield images show fluorescence complementation after transfecting split mNeonGreen2 parental cell line (smNG2-P) cells with a plasmid expressing H2B fused to mNG211. The scale bar is 10 microns.
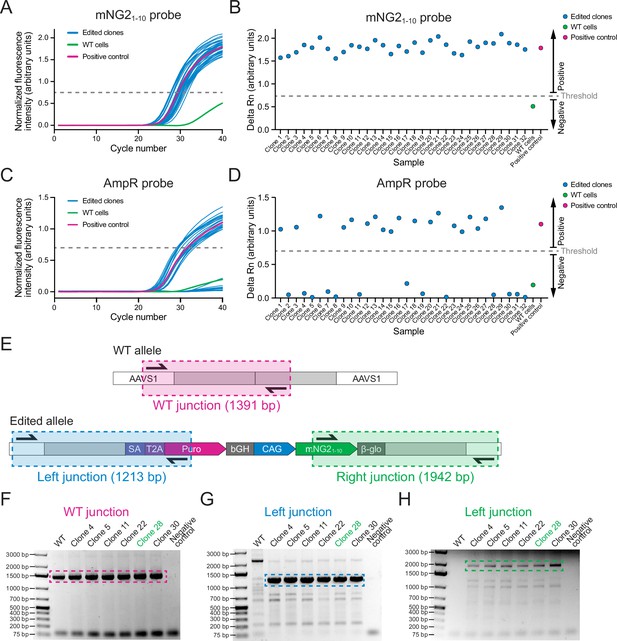
Screening of AAVS1-mNG21-10 clones.
(A) A graph shows qPCR amplification curves for the mNG21-10 probe across 32 edited clones (blue), wild-type (WT) cells (green), and a positive control (pink). (B) A graph shows the delta Rn values (increase in fluorescence over the 40 qPCR cycles) to show the presence or absence of the mNG21-10 gene in 32 clones edited clones (blue), WT cells (green), and a positive control (pink). Clones with delta Rn values above the threshold were considered positive for mNG21-10. (C) A graph shows qPCR amplification curves for the AmpR probe in 32 edited clones (blue), WT cells (green), and a positive control (pink). (D) A graph shows the delta Rn values (increase in fluorescence over the 40 qPCR cycles) to show the presence or absence of the AmpR gene in 32 edited clones (blue), WT cells (green), and a positive control (pink). Clones with delta Rn values below the threshold were considered negative for Ampicillin resistance (AmpR). (E) A schematic shows the strategy for junction PCR screening. Three sets of primers were used to amplify the WT AAVS1 allele (top, pink), and the left (bottom left, blue), and right (bottom right, green) integration junctions to verify the mNG21-10 insertion. (F–H) Gel images show the results of the junction PCR screening for WT (F), left junction (G), and right junction (H) with WT cells and six edited clones. The hatched boxes indicate the relevant DNA band. Clone 28 (green) was selected for further validation.
-
Figure 1—figure supplement 1—source data 1
Source data for Figure 1—figure supplement 1A—D.
- https://cdn.elifesciences.org/articles/92819/elife-92819-fig1-figsupp1-data1-v2.xlsx
-
Figure 1—figure supplement 1—source data 2
Folder containing the raw and annotated gel images used for Figure 1—figure supplement 1F, G, and H.
- https://cdn.elifesciences.org/articles/92819/elife-92819-fig1-figsupp1-data2-v2.zip

Validation of the split mNeonGreen2 parental cell line (smNG2-P) cell line.
(A) A bar graph shows the copy number of the mNG21-10 (green) and AmpR (pink) genes in clone 28 and wild-type (WT) cells determined by digital PCR. (B–M) Sequence chromatograms for 12 AAVS1 off-target sites in AAVS1-mNG21-10 clone 28 cells are shown below the reference sequence from the human genome. The mismatched sgRNA target and PAM sites are highlighted in red. (N) A brightfield image shows AAVS1-mNG21-10 clone 28 cells growing on an iMatrix-511 Silk-coated plate. (O) A gel image shows the mycoplasma PCR test with cells from several AAVS1-mNG21-10 clones, along with positive and negative controls. Clone 28 is highlighted in green.
-
Figure 1—figure supplement 2—source data 1
Source data for Figure 1—figure supplement 2A.
- https://cdn.elifesciences.org/articles/92819/elife-92819-fig1-figsupp2-data1-v2.xlsx
-
Figure 1—figure supplement 2—source data 2
Folder containing the raw and annotated gel images used for Figure 1—figure supplement 2O.
- https://cdn.elifesciences.org/articles/92819/elife-92819-fig1-figsupp2-data2-v2.zip
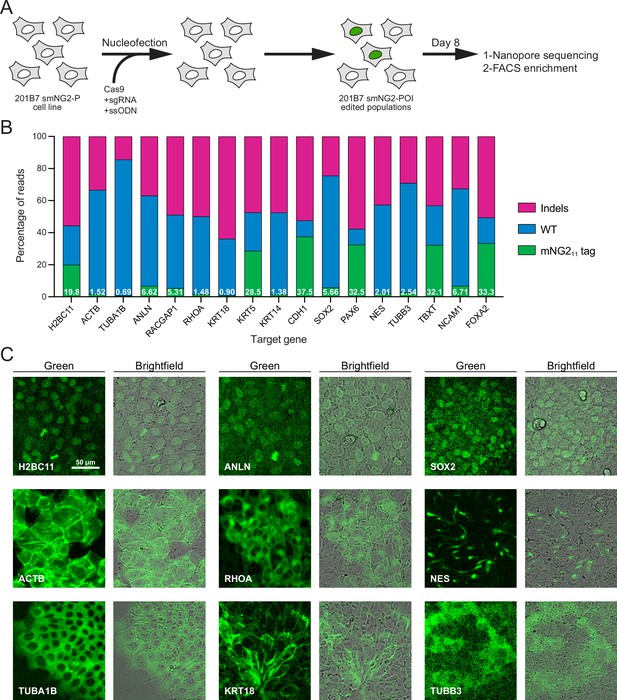
Efficient endogenous tagging with mNG211 in split mNeonGreen2 parental cell line (smNG2-P) cells.
(A) A schematic shows the workflow used to tag proteins of interest with the mNG211 fragment. smNG2-P cells were transfected with Cas9/sgRNA RNPs and a single-stranded repair template (ssODN) to integrate mNG211 at a target locus. After recovery, the edited populations were frozen and assessed by Nanopore sequencing and flow cytometry. (B) A bar graph shows the distribution of alleles (WT, indels, or mNG211-tagged) in edited populations. The percentage of mNG211 alleles is indicated in white for each gene. (C) Fluorescent and brightfield images show populations of tagged cells after enrichment by FACS as indicated. The scale bar is 50 microns.
-
Figure 2—source data 1
Source data for Figure 2B.
- https://cdn.elifesciences.org/articles/92819/elife-92819-fig2-data1-v2.xlsx
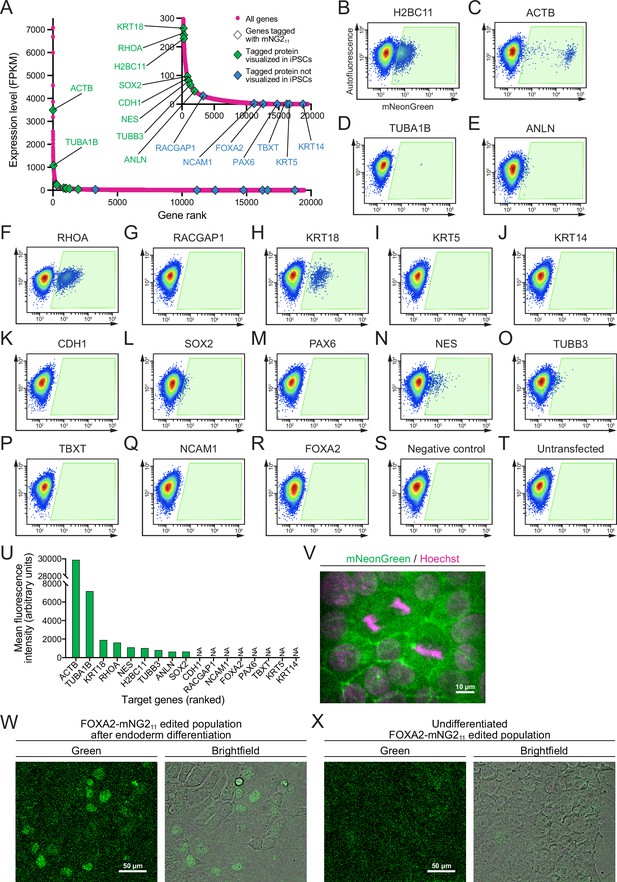
Fluorescent signal from endogenous mNG211 tags.
(A) A graph shows the expression levels of protein-coding genes in the 201B7 cell line. Genes targeted for tagging with mNG211 are indicated by triangles (green for tagged proteins that were detected and blue for tagged proteins that were not detected in induced pluripotent stem cells, iPSCs). The RNA-seq data was obtained from Iwasaki et al., 2022; GEO accession number GSE199820. (B–T) Flow cytometry plots show 17 different cell populations with smNG2 fluorescence after being edited with the mNG211 tag. A negative control transfected with pooled single-stranded repair templates (ssODNs) and scrambled sgRNAs (R), and an untransfected control (S) were used to set a gate to identify fluorescent cells (gate shown in green). (U) A bar graph shows the mean fluorescence intensity of fluorescent cells in the gates shown in (B–T). (V) Fluorescent images show the localization of smNG2-tagged E-cadherin (green) and DNA (stained with Hoechst, magenta). The scale bar is 10 microns. (W–X) Fluorescent and brightfield images show the expression of smNG2-tagged FOXA2 in the edited cell population after differentiation into endoderm (U) but not in the undifferentiated cell population (V). The scale bar is 50 microns.
-
Figure 2—figure supplement 1—source data 1
Source data for Figure 2—figure supplement 1U.
- https://cdn.elifesciences.org/articles/92819/elife-92819-fig2-figsupp1-data1-v2.xlsx
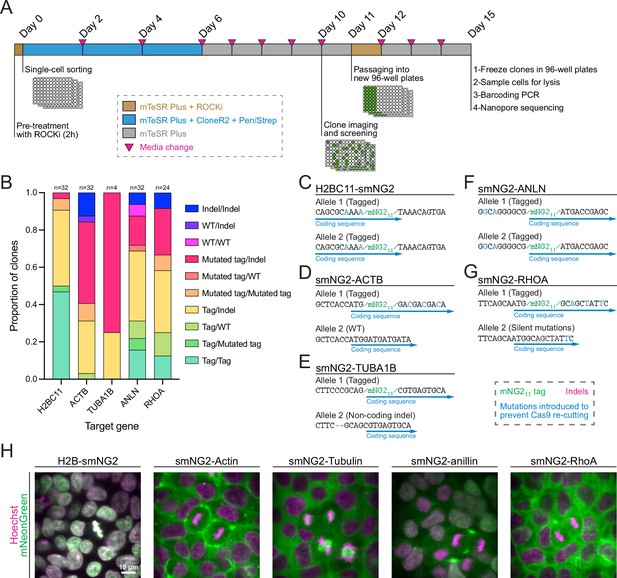
Efficient clonal isolation and screening of tagged induced pluripotent stem (iPS) cell lines.
(A) The protocol used for single-cell isolation and recovery of tagged iPSC clones is shown. Tagged cell populations were pre-treated with ROCK inhibitor Y-27632 for 2 hr before sorting individual cells into 96-well plates by FACS. Cells were kept in recovery media for 6 days, then colonies were screened on day 10 to select those for further passaging into 96-well plates on day 11. Fully-grown clones were frozen on day 15, and cells were genotyped by barcoding PCR followed by Nanopore sequencing. (B) A stacked bar graph shows the distribution of genotypes inferred from multiplexed Nanopore sequencing for isolated clones of tagged H2BC11, ACTB, TUBA1B, ANLN, and RHOA (sample size is indicated above each bar). (C–G) The genotypes of the final tagged cell lines are shown: H2BC11-smNG2 (C), smNG2-ACTB (D), smNG2-TUBA1B (E), smNG2-ANLN (F), and smNG2-RHOA (G). The target gene coding sequence is in blue, indel mutations are in red and mNG11 tag in green. (H) Fluorescent images show the localization of smNG2 (green) and DNA (stained with Hoechst; magenta) in the final tagged cell lines. The scale bar is 10 microns.
-
Figure 3—source data 1
Source data for Figure 3B.
- https://cdn.elifesciences.org/articles/92819/elife-92819-fig3-data1-v2.xlsx
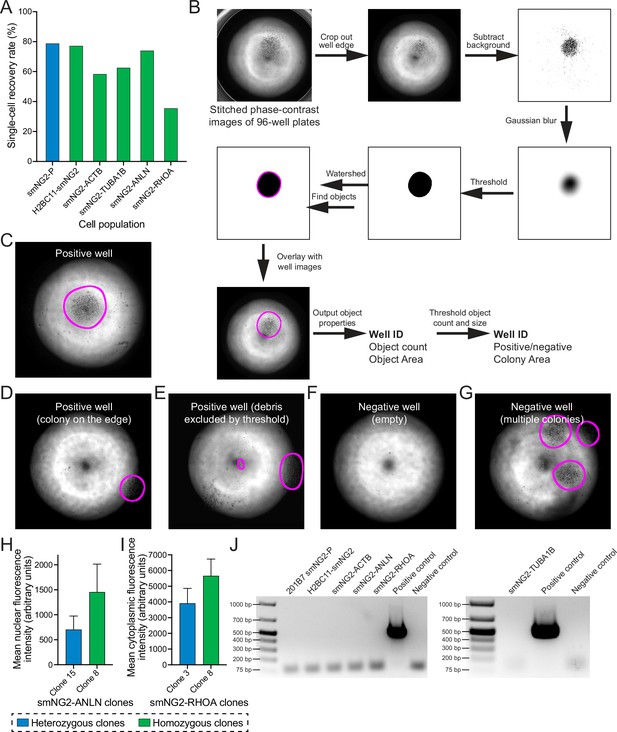
Clonal isolation and screening of tagged clones.
(A) A bar graph shows the recovery rate of different edited and wild-type (WT) cell populations after single-cell isolation by FACS. (B) The steps used by the macro to process images for colony screening in 96-well plates are shown. (C–G) Representative images of results from colony screening using the macro in B. (H) Mean nuclear fluorescence intensity of a heterozygous (shown in blue, n=120) and homozygous (shown in green, n=112) smNG2-anillin clone measured by fluorescence microscopy. (I) Mean cytoplasmic fluorescence intensity of a heterozygous (shown in blue, n=103) and homozygous (shown in green, n=101) smNG2-RhoA clone measured by fluorescence microscopy. (J) Gel images show the results of the mycoplasma PCR test for the final mNG211-tagged cell lines compared to positive and negative controls. The bar graphs in H and I show the average with standard deviation error bars.
-
Figure 3—figure supplement 1—source data 1
Source data for Figure 3—figure supplement 1A, H, I.
- https://cdn.elifesciences.org/articles/92819/elife-92819-fig3-figsupp1-data1-v2.xlsx
-
Figure 3—figure supplement 1—source data 2
Folder containing the raw and annotated gel images used for Figure 3—figure supplement 1J.
- https://cdn.elifesciences.org/articles/92819/elife-92819-fig3-figsupp1-data2-v2.zip

Image restoration for live imaging and quantitative measurements in induced pluripotent stem cells ( iPSCs).
(A) A graph shows the signal-to-noise ratio measured from low- (pink) and high-exposure (blue) images of the tagged iPS cell lines as indicated (n≥7 for each condition). (B) A schematic shows the training and application of the Content-Aware image REstoration (CARE) neural network for image restoration. The neural network developed by Weigert et al., 2018 was trained on sets of low- and high-exposure images for each tagged cell line and used to restore timelapse movies. (C–G) Comparisons of raw (Input; top panel) and restored timelapse images (Network; bottom panel) by the CARE neural network are shown for iPSCs expressing smNG2-anillin (C), smNG2-RhoA (D), H2B-smNG2 (E), smNG2-Tubulin (F), and smNG2-actin (G) undergoing cytokinesis (smNG2 in green; DNA stained with Hoechst in magenta). The scale bars are 10 microns, and time is relative to anaphase onset (00:00). The box plot in A shows the median line, quartile box edges and the minimum and maximum value whiskers.
-
Figure 4—source data 1
Source data for Figure 4A.
- https://cdn.elifesciences.org/articles/92819/elife-92819-fig4-data1-v2.xlsx
Comparison of raw (left) and restored (right) timelapse images of a smNG2-ANLN induced pluripotent stem (iPS) cell undergoing cytokinesis.
smNG2-anillin is shown in green and the DNA (stained with Hoechst) is shown in magenta. Images were acquired every minute and played at four frames per second (240 x real-time). Time is shown relative to anaphase onset (00:00). The scale bar is 10 microns. Still, images are shown in Figure 4C.
Comparison of raw (left) and restored (right) timelapse images of a smNG2-RHOA induced pluripotent stem (iPS) cell undergoing cytokinesis.
smNG2-RhoA is shown in green and the DNA (stained with Hoechst) is shown in magenta. Images were acquired every minute and played at four frames per second (240 x real-time). Time is shown relative to anaphase onset (00:00). The scale bar is 10 microns. Still, images are shown in Figure 4D.
Comparison of raw (left) and restored (right) timelapse images of a H2BC11-smNG2 induced pluripotent stem (iPS) cell undergoing cytokinesis.
Images were acquired every minute and played at four frames per second (240 x real-time). Time is shown relative to anaphase onset (00:00). The scale bar is 10 microns. Still, images are shown in Figure 4E.
Comparison of raw (left) and restored (right) timelapse images of a smNG2-TUBA1B induced pluripotent stem (iPS) cell undergoing cytokinesis.
smNG2-Tubulin is shown in green and the DNA (stained with Hoechst) is shown in magenta. Images were acquired every minute and played at four frames per second (240 x real-time). Time is shown relative to anaphase onset (00:00). The scale bar is 10 microns. Still, images are shown in Figure 4F.
Comparison of raw (left) and restored (right) timelapse images of a smNG2-ACTB induced pluripotent stem (iPS) cell undergoing cytokinesis.
smNG2-Actin is shown in green and the DNA (stained with Hoechst) is shown in magenta. Images were acquired every minute and played at four frames per second (240 x real-time). Time is shown relative to anaphase onset (00:00). The scale bar is 10 microns. Still, images are shown in Figure 4G.
Comparison of raw (left) and restored (right) timelapse images of a smNG2-ACTB induced pluripotent stem (iPS) cell showing colony-scale re-arrangements of the actin network after cytokinesis.
smNG2-Actin is shown in green and the DNA (stained with Hoechst) is shown in magenta. Images were acquired every minute and played at four frames per second (240 x real-time). Time is shown relative to anaphase onset (00:00). The scale bar is 10 microns. Still, images are shown in Figure 5—figure supplement 1A.

Cytokinesis is uniquely regulated in induced pluripotent stem cells (iPSCs).
(A) Schematics show the core pathways regulating contractile ring assembly and ingression (left) and their localization inside a late anaphase cell (right). (B) A schematic (left) shows how the duration of ingression was measured in anillin-tagged iPSCs (right; n=39). (C) A schematic (left) shows how the ratio of cortical to cytosolic protein was measured in metaphase cells for smNG2-anillin, smNG2-RhoA, and smNG2-actin (right; n=10 for each). (D) A schematic (left) shows the location and timing of the linescans used to plot the fluorescence intensity along the cortex in single iPSCs for smNG2-anillin (center) and smNG2-RhoA (right). Timepoints are shown in different colors as indicated in the scale. (E) A schematic (left) shows the location of the linescans used to plot the intensity of fluorescence along the cortex at the onset of furrowing for smNG2-anillin (center) and smNG2-RhoA (right; n=10 for each). (F) A schematic (left) shows how the breadth of protein localization at the equatorial cortex at furrow initiation was calculated for smNG2-anillin and smNG2-RhoA (n=10 for each). (G) Fluorescent images show the location of DNA (left; stained with Hoechst) and smNG2-Tubulin (gray scale) in a cell. For tubulin, a lower focal plane (center) is shown to highlight astral microtubules (indicated by pink arrows), and a higher focal plane (right) is shown to highlight the central spindle. The scale bar is 10 microns. (H) A schematic (top) shows the location of the linescan used to plot the fluorescence intensity along the midzone of a cell expressing smNG2-tubulin in anaphase (bottom). (I) A schematic shows a comparison of the conventional view of cytokinesis (HeLa cell; left) and an iPS cell (iPSCs; right). HeLa cells have a strongly enriched central spindle, which keeps the equatorial of active RhoA narrow, and astral microtubules that extend all the way to the cortex and restrict the localization of anillin to the same zone as RhoA. iPSCs have a weakly enriched central spindle, resulting in a broader zone of active RhoA, and prominent astral microtubules that keep anillin in a narrow equatorial zone. Box plots in B, C, and F show the median line, quartile box edges and the minimum and maximum value whiskers. Statistical significance was determined by Brown-Forsythe and Welch’s ANOVA follow by Dunnett’s T3 test for C and Welch’s t-test for F (ns, not significant; *p≤0.05; **p≤0.01; ***p≤0.001; ****p≤0.0001).
-
Figure 5—source data 1
Source data for Figure 5B, C, D, E, F and H.
- https://cdn.elifesciences.org/articles/92819/elife-92819-fig5-data1-v2.xlsx

Protein localization in induced pluripotent stem cells (iPSCs) during cytokinesis.
(A) Timelapse images show a cell expressing smNG2-actin during cytokinesis. The input images are shown above the same images after restoration by the Content-Aware image REstoration (CARE) neural network. The mNeonGreen2 (mNG2) signal is shown in green and the DNA is stained with Hoechst (magenta). The scale bar is 10 microns and times are shown relative to anaphase onset (00:00). (B) A schematic (left) shows how the breadth of protein localization at the equatorial cortex relative to cortex length was calculated for smNG2-anillin and smNG2-RhoA (right; n=10 for each). The box plot in B shows the median line, quartile box edges and the minimum and maximum value whiskers. Statistical significance was determined by Welch’s t-test (ns, not significant; *p≤0.05; **p≤0.01; ***p≤0.001; ****p≤0.0001).
-
Figure 5—figure supplement 1—source data 1
Source data for Figure 5—figure supplement 1B.
- https://cdn.elifesciences.org/articles/92819/elife-92819-fig5-figsupp1-data1-v2.xlsx
Tables
Reagent type (species) or resource | Designation | Source or reference | Identifiers | Additional information |
---|---|---|---|---|
Strain, strain background (Escherichia coli) | DH5α | Genome Canada | ||
Strain, strain background (Escherichia coli) | ER2925 | Genome Canada | Methylase-deficient strain | |
Cell line (Homo sapiens) | 201B7 | ATCC | ACS-1023 | |
Cell line (Homo sapiens) | smNG2-P | This paper | ||
Antibody | Mouse monoclonal anti-NANOG | DSHB | PCRP-NANOGP1-2D8 | 1:25 dilution |
Antibody | Mouse monoclonal anti-OCT3/4 | Santa Cruz Biotechnology | sc-5279 | 1:20 dilution |
Antibody | Mouse monoclonal Alexa488-conjugated anti-TRA-1–60 | STEMCELL Technologies | 60064AD | 1:20 dilution |
Antibody | Rabbit polyclonal Alexa488-conjugated anti-mouse | Thermo Fisher Scientific | A-11059 | 1:400 |
Antibody | Mouse monoclonal anti-PAX6 | DSHB | PAX6 | 1:45 |
Antibody | Mouse monoclonal anti-NCAM | DSHB | 5.1H11 | 1:240 |
Antibody | Mouse monoclonal PE-conjugated anti-FOXA2 | BD Biosciences | 561589 | 1:50 |
Recombinant DNA reagent | pNCS-mNeonGreen | Allele Biotechnology | ABP-FP-MNEONSB | |
Recombinant DNA reagent | pAAVS1-P-CAG-GF | Oceguera-Yanez et al., 2016 | Addgene #80491 | |
Recombinant DNA reagent | pAAVS1-P-CAG-mNG21-10 | This paper | Addgene #206042 | |
Recombinant DNA reagent | pH2B-mNG211 | This paper | Addgene #206043 | |
Sequence-based reagent | sgRNA | Sigma-Aldrich | Listed in Supplementary file 3 | |
Sequence-based reagent | sgRNA | Synthego | Listed in Supplementary file 3 | |
Sequence-based reagent | ssODN | BioCorp | Listed in Supplementary file 4 | |
Sequence-based reagent | Primers | Thermo Fisher Scientific | Listed in Supplementary file 5 and 6 | |
Sequence-based reagent | Hydrolysis probes | IDT | Sequences listed in the Materials and Methods | |
Peptide, recombinant protein | Cas9-eGFP | STEMCELL Technologies | 76006 | |
Peptide, recombinant protein | Cas9 | Synthego | SpCas9 2NLS Nuclease | |
Commercial assay or kit | P3 Nucleofection kit | Lonza | V4SP-3096 | |
Commercial assay or kit | STEMdiff Trilineage Differentiation Kit | STEMCELL Technologies | 05230 | |
Commercial assay or kit | QuickExtract | Biosearch Technologies | QE0905T | |
Commercial assay or kit | Ligation Sequencing Kit V14 | Oxford Nanopore Technologies | SQK-LSK114 | |
Commercial assay or kit | NEBNext Companion Module | New England Biolabs | E7180S | |
Commercial assay or kit | RNaseP reference assay | Thermo Fisher Scientific | 4403328 | |
Commercial assay or kit | QuantStudio 3D Digital PCR Master Mix V2 | Thermo Fisher Scientific | A26358 | |
Commercial assay or kit | QuantStudio 3D Digital PCR 20 K Chip Kit V2 | Thermo Fisher Scientific | A26316 | |
Chemical compound, drug | CloneR2 | STEMCELL Technologies | 100–0691 | Single-cell recovery reagent |
Chemical compound, drug | NU7441 | Tocris Bioscience | 3712 | NHEJ inhibitor |
Chemical compound, drug | SCR7 | Xcess Biosciences | M60082 | NHEJ inhibitor |
Chemical compound, drug | Hoechst 33342 | Thermo Fisher Scientific | H1399 | |
Software, algorithm | CARE | Weigert et al., 2018 | https://github.com/CSBDeep/CSBDeep; Schmidt et al., 2023 | Version 0.7.2 |
Software, algorithm | Fiji | NIH | https://imagej.net/software/fiji/ | Version 2.3 |
Software, algorithm | CRISPResso2 | Clement et al., 2019 | https://github.com/pinellolab/CRISPResso2; Pinello Lab, 2024 | Version 2.2.12 |
Other | 35 mm polymer coverslip dish | Cellvis | D35-20-1.5P | Coverslips for live imaging |
Additional files
-
Supplementary file 1
Characterization and validation of the smNG2-P cell line.
The results from the validation and quality control steps carried out on the smNG2-P cell line are listed.
- https://cdn.elifesciences.org/articles/92819/elife-92819-supp1-v2.xlsx
-
Supplementary file 2
AAVS1 off-target sites sequencing in 201B7 smNG2-P cells.
The top off-target sites for the AAVS1 sgRNA are listed, along with the results of sequencing these sites in the split mNeonGreen2 parental (smNG2-P) cell line compared to wild-type (WT) cells.
- https://cdn.elifesciences.org/articles/92819/elife-92819-supp2-v2.xlsx
-
Supplementary file 3
List of sgRNAs used in this study.
The sequences of all sgRNAs used in this study are listed.
- https://cdn.elifesciences.org/articles/92819/elife-92819-supp3-v2.xlsx
-
Supplementary file 4
List of single-stranded repair templates (ssODNs) used for endogenous tagging with mNG211.
The sequences of the single-stranded oligonucleotides used as repair templates to integrate mNG211 at various loci are shown. For each oligonucleotide, the homology arms are shown in blue, the mNG211 tag in green, the protein linker in purple and mutations designed to remove homology and prevent Cas9 re-cutting in red.
- https://cdn.elifesciences.org/articles/92819/elife-92819-supp4-v2.xlsx
-
Supplementary file 5
List of genotyping primers.
The primers used to amplify and sequence each edited locus are listed.
- https://cdn.elifesciences.org/articles/92819/elife-92819-supp5-v2.xlsx
-
Supplementary file 6
List of barcoding primers.
The primers used to add unique barcodes onto locus-specific amplicons for pooled Nanopore sequencing are shown.
- https://cdn.elifesciences.org/articles/92819/elife-92819-supp6-v2.xlsx
-
Supplementary file 7
List of live imaging parameters.
For each cell lines imaged, the laser power and exposure time used to acquire low- and high-exposure images are listed. These parameters were used to acquire the neural network training dataset, and the low-exposure settings were used to acquire timelapse images for restoration.
- https://cdn.elifesciences.org/articles/92819/elife-92819-supp7-v2.xlsx
-
MDAR checklist
- https://cdn.elifesciences.org/articles/92819/elife-92819-mdarchecklist1-v2.docx